Optical Networks explained
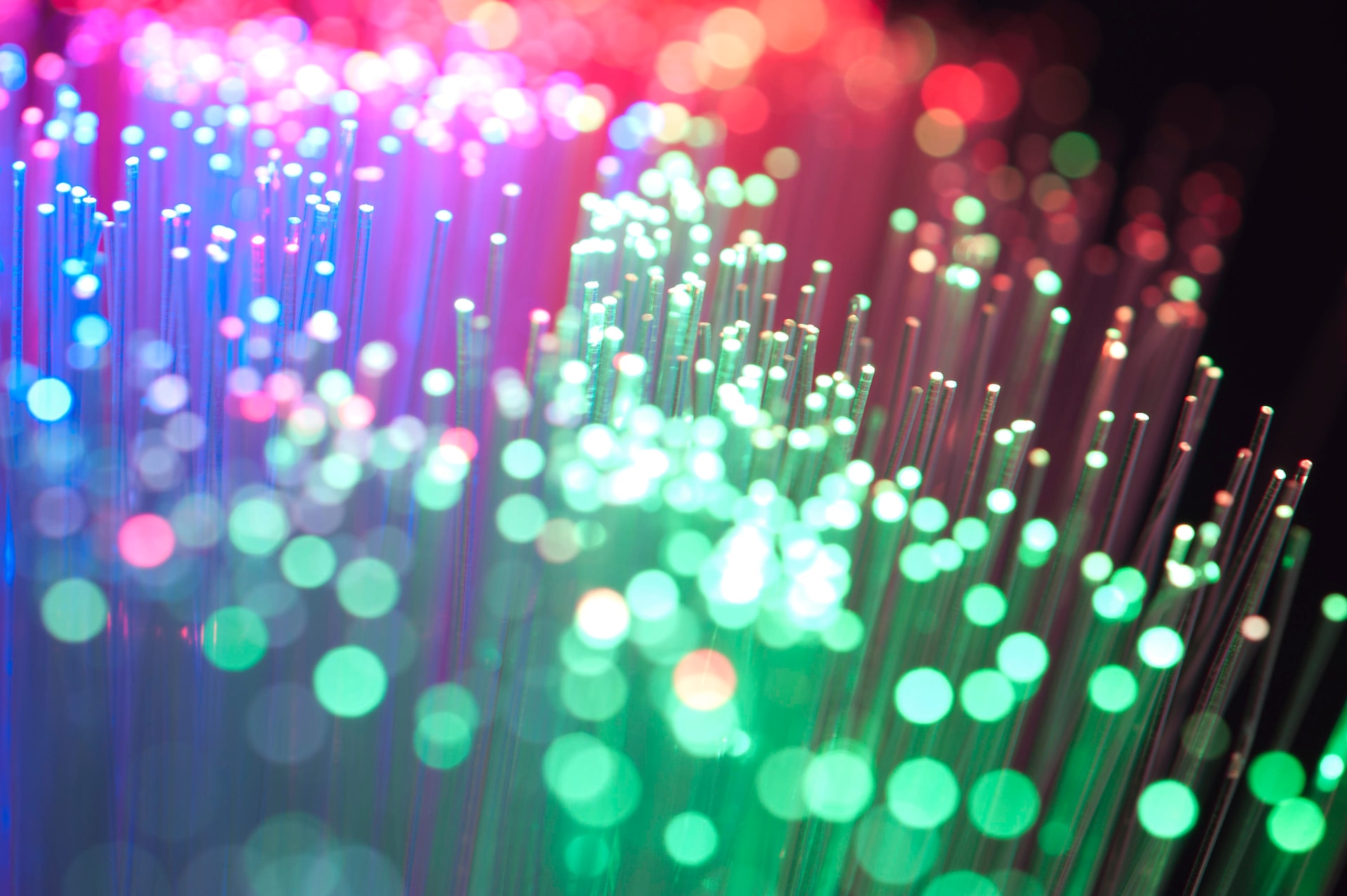
There are primarily three physical media used for transmitting network information today: copper cabling, first used for the telegraph in the 1820s and still the most prevalent cabled medium; radio spectrum, first used by Marconi in 1901, and the fastest growing medium today; and fiber optic cables, used since 1977 when General Telephone and Electronics sent the first live telephone traffic through fiber optics in Long Beach, California.
Of the three media, fiber optics has the greatest potential to handle the majority of our networking needs, with radio providing mobile access. Optical transport networks are based on the use of glass strands of optical fiber, each no thicker than a human hair, that can transmit light pulses, and thus information, with practically no limits on distance, or capacity. In this article, we will look at fiber optic networks, how they work, and recent technology advances.
The history of optical transport networks is about progressively replacing networks based on transmitting electrical signals over copper wire. Copper-based cabling was originally used by companies offering telegraph and telephone services. Optical fiber, although known since the early 20th century, only became a viable replacement for copper in the 1980s and 1990s. Often touted for its almost limitless information-carrying capacity, its energy efficiency may be becoming its most important characteristic.
Fiber vs copper
The decision whether to use copper or fiber comes down to a trade-off between the expense of the technology vs. the amount of information it can carry and the energy it saves. This calculation has traditionally meant that fiber optics were used for long-haul transmission networks (sub-sea and terrestrial), and more recently, for metropolitan area networks. As we will see, however, innovations and refinements to optical technology mean that the trend is toward the use of fiber optics in more and more local applications: to the home, and to interconnect data centers.
How is data transmitted on optical fiber?
Optical fibers are long strands of specially formulated glass filaments through which light can be sent over long distances. The transmission is done using lasers that send pulses of light through the glass. Each pulse represents either a “zero” or a “one”; the binary data bits that encode all our modern communications, applications, and connectedness.
Although conceptually simple, several difficult hurdles had to be overcome to make this a reality. The first of these hurdles was signal attenuation caused by noise or imperfections within the glass. This was solved by Corning Glass in 1975, which pioneered the manufacture of ultra-pure, low-loss, fiber optic cables. From this point, fiber optic communications were born, with the first optical networks being commercially used for telephone traffic starting in the late 1970s.
Next came optical amplification. No matter how little noise is created by the glass fiber, attenuation and dispersion of the light signal are always present to some degree. Without amplification, a light signal will only travel so far before it becomes unreadable – the distance varies based on several factors, but at some point, in most network spans, amplification is required, typically after 80 to 100 km.
Initially, the only way to amplify the signal was to convert the light signal back to an electrical signal, then electrically re-transmit it, and then convert it back to light. These optical-electrical-optical (OEO) repeaters were costly, power hungry, and used lots of space. They were also highly inefficient, especially when amplifying multiple channels at the same time. Optical amplifiers, which avoid the conversion to electrical, require less power and, more importantly, one amplifier can amplify many channels at once, which became very important when multiplexing many channels on one fiber.
The ability to transmit multiple light signals in parallel down a single fiber is called wave division multiplexing (WDM) and started being widely deployed in 1995. Originally lasers used one color of light to send their pulses, but in the 1980s, researchers at Nokia Bell Labs and elsewhere found a way to send multiple colors or wavelengths of light through the same glass fiber. Thus, a single fiber, instead of carrying one channel on one color, could carry multiple channels in both directions on multiple, different colors. A later development, dense WDM (DWDM), can transmit 40 or 80 duplex channels or colors, thus increasing the information capacity of a single fiber by 40 or 80 times.
Combined, optical amplification and WDM created massive, order of magnitude efficiencies in information carrying capacity, which made fiber optics less expensive than copper-based electrical transmission over practically all distances. Passive optical networks (PON) have increasingly been used since the 1980s to deliver fiber-to-the-home broadband access to the home and enterprise market. In the 1990s, fiber optics began to be used in enterprise local area networks (LANs) to connect Ethernet switches and IP routers. And since the 1990s, fiber optics using DWDM have come to dominate long-haul and metropolitan transport networks, especially as internet traffic exploded.
How coherent transmission enabled massive scaling of optical networks
Along with better glass fiber, there are numerous technologies required to continue scaling the data capacity of the transmitting lasers at one end, and the receivers at the other end of the glass strand. These have been an object of continuous research and development by Nokia Bell Labs and other researchers in universities and industry over the last 50 years.
The last few decades have seen enormous scaling in data transmission speeds using coherent transmission techniques. Combining the technology of digital signal processors (DSPs) with the application of phase modulation techniques (also common in cellular radio networks) to optical signals, coherent technology has enabled a 100-fold increase in wavelength speed since it was first introduced a decade ago.
Before describing coherent transmission, let’s look at how all optical transport networks transmitted information before. It was done by on-off-keying (OOK), which essentially turned a light signal on to transmit a “one, or off, to transmit a “zero”. This sounds simple, and it was used for a long time, but there is a limit to how fast you can turn a laser on and off again. Workarounds were developed but they only ever offered incremental speed increases, and the limit for OOK transmission was achieved at a data rate of 10 Gb/s per wavelength (OC-192) in the late 1990s. This was still significant and led to the massive network upgrades that happened from the late-1990s until circa 2010.
It was the advent of coherent transmission, however, that provided the ground-breaking leap to what is now the de facto optical transmission technology for 400G, 600G, 800G and soon terabit solutions.
Instead of turning the laser on or off, coherent transmission makes it possible to use phase modulation to create a binary signal. Light waves are like other waves across the radio spectrum, and can be modulated to be bigger or smaller, or to stop and start at different times. By changing their phase and amplitude, it is, for instance, possible to indicate a 0 with a small wave and a 1 with a big wave. Using phase modulation, you can move the wave forward or backward, adding further information based on different points in the wave form, that is, not just the crest and the trough. You can also change the vertical and horizontal polarization of the light to create more or less intensity.
Quadratic amplitude modulation (QAM) uses all these techniques together. Coherent transmission uses a variation of QAM called quadratic phase shift keying (QPSK) to transmit much more information than OOK. Early coherent systems used QPSK at 32 GBaud meaning the signal state changes 32 billion times a second. The signal state can be 8, 16, 32 or 64 QAM. For instance, at 64 QAM at 64 GBaud (64/64), you could transmit 600 billion bits per second or 600 Gbit/s on a single channel of light. Repeated across 128 channels, today’s fiber optic networks can transmit close to 80 terabits per second of data, over hundreds of kilometers of fiber, without electrical repeaters.
How do pluggable digital coherent optics (DCO) take coherent to the network edge?
As optical transmission technologies progressed, the optical components that transmit and detect the light, such as lasers and detectors, have also been miniaturized to the point that they can fit into small, low power and pluggable packages. This makes it possible to plug all the needed opto-electronic devices as a single module into an optical interface port in a router. Pluggable transceivers have the advantage that if the transceiver fails, it is a simple plug replacement instead of the whole line card. They also allow a range of optics with different characteristics to be used interchangeably, without having to design specific line-cards for each. In either case, it means that fiber optics have become affordable enough that they can be deployed for a wide variety of uses besides metro and long-haul transmission, and they are replacing copper-based cabling everywhere from the data center LAN to last mile access and short- and medium-haul applications.
1Even at the micro level. Many industry initiatives are looking to replace copper wire on circuit boards with optical fiber circuits that interconnect silicon ICs to each other. This is referred to as “on-board optics” (OOB) or “co-packaged optics” (CPO).
In carrier networks, these new pluggable coherent transceivers are now used for router interfaces at speeds up to 400 Gbit/s and will soon scale to even higher speeds. Nokia’s Coherent Routing solution, for instance, uses pluggable coherent transceivers with its high-speed routers to enable direct router-to-router data center interconnection or other metro-level applications. This saves power by bypassing dedicated optical transport systems used in fiber optic transmission networks to do the transmission and receiving.
What is the future of optical transport networks?
The most advanced optical transmission technology is operating within a decibel (dB) of the Shannon limit, originally described by Claude Shannon of Bell Labs as the maximum rate of error-free data that a channel can carry. Overcoming this physical limitation will require significant innovations to enable optical transport networks to continue meeting our growing needs in the future. Research in this area ranges from hollow core fibers to the use of machine learning to improve signal processing. Nokia Bell Labs is at the forefront of this work and our research and innovations will continue to find their way into Nokia products as we build the networks of the future.
Read more
About Nokia
We create technology that helps the world act together.
As a trusted partner for critical networks, we are committed to innovation and technology leadership across mobile, fixed and cloud networks. We create value with intellectual property and long-term research, led by the award-winning Nokia Bell Labs.
Adhering to the highest standards of integrity and security, we help build the capabilities needed for a more productive, sustainable and inclusive world.
Media Inquiries:
Nokia
Communications
Phone: +358 10 448 4900
Email: press.services@nokia.com