MIMO is set to transform optical networks the same way it revolutionized wireless
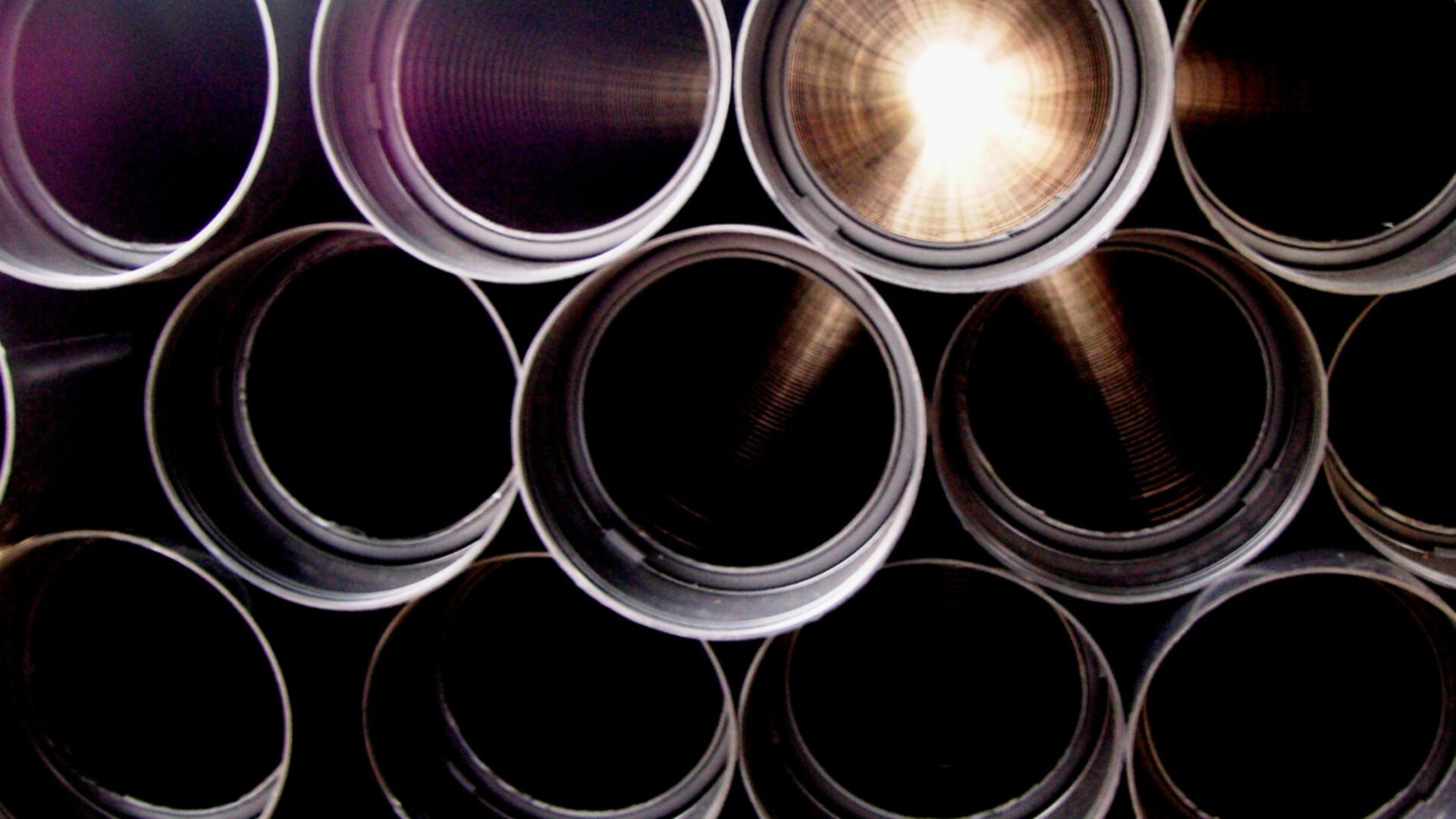
If I were the say the word “MIMO,” the first image to pop into your head would likely be of a wireless network. Multiple-input-multiple-output technology has been an essential tool in WiFi and cellular systems since the days of HSPA+. It’s easy to see why MIMO has had such a lasting impact on wireless. By using the same frequencies to send information over different paths, we are able to increase the capacity of the wireless channel while sharing spectrum effectively across many users.
What you may not know, however, is that MIMO is set to make a similar impact on optical networks. Unlike cellular and WiFi systems, fiber transmissions have a predefined route, but within the physical medium of fiber we can create multiple paths, or modes, through which we can send information. In fact, MIMO is already widely used in long-distance fiber-optic communication through polarization. By making use of the two polarization channels we are sending information over the same frequency in the same fiber core along separate paths – in effect utilizing 2x2 MIMO.
What’s exciting, though, is that Nokia Bell Labs is now doing the fundamental research in space division multiplexing (SDM) that will allow us to expand optical MIMO techniques beyond 2x2 polarization systems. We will be able to create fiber systems that will reuse the same wavelength of light dozens of times, providing new ways to significantly increase communication capacity and cost-effectively meet the world’s insatiable demand for data.
Multimode fiber
In fiber optics, modes are specific light patterns that propagate through fiber unchanged. Today for long-distance communication we typically use fibers that support exactly one mode. But we can increase the number of modes supported in a single strand of fiber by changing its dimensions and the material composition of its core, allowing us to send multiple light patterns over the same optical wavelength. The problem is that while propagating over distance, those distinct light patterns will mix with one another due to tiny fiber imperfections introduced in the manufacturing process. What starts out at the transmitter as a series of separate light patterns becomes a single indecipherable pattern by the time it reaches the receiver many kilometers away.
This is where MIMO comes in. By using the same MIMO techniques that separate wireless transmissions that mix as they propagate through air, we can unravel the intertwined light patterns that mix as they propagate through fiber. By preserving the distinct nature of each mode we can rapidly scale up the number of modes in a single fiber core. Our research has produced multimode cores either through widening the diameter of the optical core or by creating higher-density optical cores. The result has been as many as 45 modes in a single core over the most commonly used optical wavelengths. And as each mode can support two polarization channels, we’ve effectively created 90x90 MIMO systems! That represents a 45X increase in capacity over today’s polarized single-mode systems.
The benefits of these MIMO techniques are readily apparent. Deploying multimode fiber has only a marginally higher cost structure than single-mode fiber, but the capacity gains are far from marginal. In scenarios where there are severe size constraints – such as within conduits or undersea cables – service providers can boost capacity without increasing the number of fibers deployed. And in the case of multi-fiber deployments, operators could exploit multimode many times over – gaining its capacity boost for every single additional fiber installed. Meanwhile, many of today’s optical components such as optical amplifiers, filters or isolators can be relatively easily modified to support multiple modes.
Multicore fiber
While we can create multiple modes by developing better fiber, we can alternatively produce a similar result by running multiple cores alongside one another in a single fiber strand. This is known as multicore fiber. Multicore, however, suffers from many of the same problems as multimode. As distances increase, light from the individual cores bleed into their neighboring cores creating garbled transmissions. We can solve this problem by spacing the parallel cores apart, but that’s hardly an efficient way to manufacture fiber systems. Or we could embrace this interference – or “coupling” – between cores and treat the multicore system as if it were a multimode system. Then we would use the same MIMO techniques to separate out the individual transmission patterns.
These coupled-core multicore fibers are very advantageous as they produce very similar properties and performance among all transmitted modal channels. Additionally, the strong coupling mitigates the nonlinear effect – a data-rate limiting factor in all fiber transmission – making multicore transmission performance superior to that of an equal number of single core systems. Finally, the MIMO techniques used in coupled-core fibers require significantly fewer processing resources than in multimode fibers, greatly reducing the expense of building multicore systems.
Multicore fibers are of particular interest to submarine cable manufacturers, as they increase the number of spatial channels – and thus capacity – of a single undersea cable without increasing the diameter of the cable or requiring any additional power. Those two factors are of enormous importance to intercontinental communications providers, which need to physically install and provide power to thousands of kilometers of cable for a single transoceanic link. So far we have been able to demonstrate that four to five uncoupled cores can fit in a standard-diameter fiber suitable for undersea cables, but by utilizing MIMO techniques we have managed to squeeze as many as 7 cores into the same physical dimensions. We think as many as 19 are possible in the future, which would represent a 19X increase in capacity over today’s single-core systems.
What’s next?
While our experiments with MIMO in multimode and coupled-core fibers clearly show that the solution is technically viable, we still need to conduct additional research on the implementation of real-time MIMO processing and optical components that support the added complexity of these new SDM advances.
But once we overcome those obstacles, the potential for MIMO in fiber transmission is enormous. The properties demonstrated in coupled-core fibers show particularly high promise in long-distance transport, potentially achieving transmission distances of more than 12000 km, which would bridge even the longest transpacific routes. Conversely multimode fibers show great potential in powerful parallel short-range links (10 km to 100 km). We have recently demonstrated multiplexers that could support up to 1000 distinct modes in a single core. That’s quite the improvement over the single-mode fiber most commonly used today.
What this all translates to is enormous gains in capacity, and those gains couldn’t come at a more opportune time. While Nokia Bell Labs is on the leading edge of spectral efficiency research, we are rapidly approaching the limit to home much capacity we can pack into a single wavelength of light. We can no longer achieve order-of-magnitude performance gains in single-mode fiber transmission rates. Consequently these new lines of inquiry into more modes, more cores and applying the techniques of MIMO to optical transmission will produce the next order-of-magnitude gains that the world has not only come to expect, but demand.