Revolutionizing Wi-Fi with L4S: A Low-Latency Future
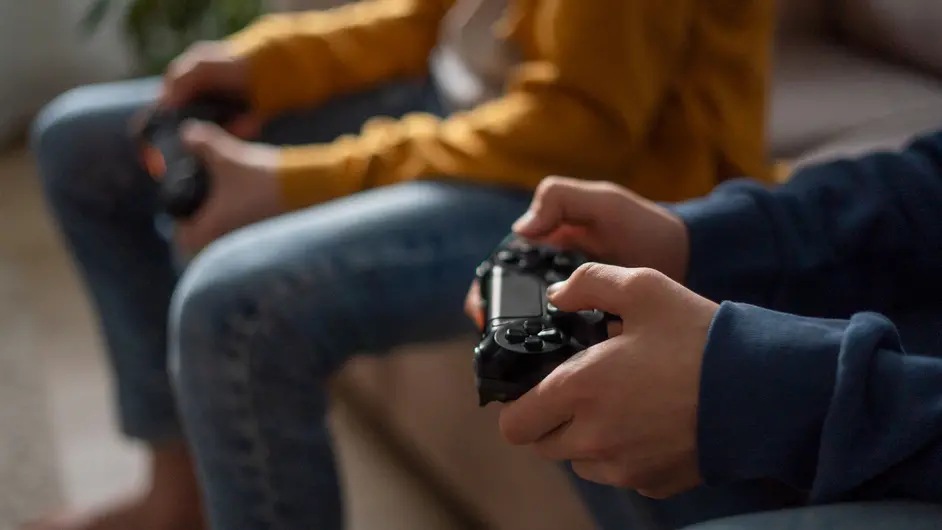
In January 2023, the Internet Engineering Task Force (IETF) released key Request for Comments (RFCs) that marked a significant step forward for Low Latency Low Loss Scalable Throughput (L4S) technology. L4S seeks to improve end-to-end network performance by addressing queuing delay, the single largest and most frequently overlooked cause of latency and latency variation, reducing it to near-zero values By using packet marking instead of packet delaying and dropping for controlling application data rates, L4S offers an unrivalled experience responsiveness with near-zero delay and packet loss.
After being the first to demonstrate L4S over Wi-Fi in 2019, Nokia Bell Labs is excited to explore L4S's transformative potential in creating a low-latency future for Wi-Fi networks. In this blog, we will explain the constraints of the Wi-Fi medium access control (MAC) layer, how L4S can overcome them to significantly reduce Wi-Fi latency under congestion, and how L4S and Wi-Fi multimedia quality of service (WMM) can work together to produce a seamless low-latency experience. With these advancements, Wi-Fi networks will be able to flawlessly support even the most demanding real-time applications like cloud gaming, video conferencing, and extended reality (XR).
L4S's impact on Wi-Fi performance
L4S has the potential to drastically reduce latency during periods of traffic congestion on Wi-Fi networks. In Figure 1, our testing with Nokia Wi-Fi beacons demonstrated that L4S could reduce latency by more than a factor of 10, from hundreds of milliseconds to just around 10 milliseconds. This significant reduction is critical for real-time applications, such as video conferencing, online gaming, XR, and other latency-sensitive tasks, where low latency is not just desirable but essential.
Figure 1: Measurement of the latency experienced by L4S (blue) and non-L4S (orange) traffic running over a fully congested Wi-Fi link. “PXX” refers to the percentiles (e.g., P99 is the 99th percentile, meaning that 99% of the packets experience a latency smaller than the depicted value).
However, while L4S can reduce queuing delay, it is not a complete solution. The Wi-Fi MAC layer, which manages when devices can transmit their data, remains a significant source of latency and jitter. Even with L4S enabled, the MAC layer can introduce delays that exceed the 10-millisecond threshold, especially as the number of connected devices increases. To truly harness the power of L4S, further optimization of the Wi-Fi MAC layer is essential.
Overcoming Wi-Fi MAC layer limitations
The Wi-Fi MAC layer manages access to the shared wireless channel, serving as a coordination mechanism for data transmission and determining when devices can transmit data over the Wi-Fi link. Packets arriving at a Wi-Fi device are temporarily held up in a buffer while the Wi-Fi MAC contends to access the shared Wi-Fi channel to forward them.
Even though L4S drastically reduces Wi-Fi latencies by eliminating queuing latency, it cannot overcome latency caused by a Wi-Fi scheduler that blocks transmission of packets for intervals easily exceeding 10 milliseconds, illustrated in Figure 2. We must optimize the Wi-Fi MAC layer itself to minimize the delay it introduces. Without these optimizations, the MAC layer will continue to be a significant source of latency and jitter, limiting the full potential of L4S technology.
Figure 2: The Wi-Fi MAC controls access to the wireless link and can temporarily halt packets, including L4S packets, until they can be transmitted.
Multimedia QoS (WMM) enhances Wi-Fi
Introduced in 2005, Wi-Fi Multimedia (WMM) quality of service prioritizes various types of network traffic, including voice, video, and best-effort data to improve the user's experience. WMM assigns higher scheduling priority to certain categories of traffic to ensure they receive the necessary data rate and reduced latency. As such, it is a convenient tool that can be used to influence the behavior of the Wi-Fi MAC. While WMM has proven effective for traditional use cases, integrating it with L4S traffic requires a more nuanced approach.
Real-time applications, such as cloud gaming, video conferencing, and extended reality (XR), are highly sensitive to jitter—variations in latency. For example, real-time video at 60 frames per second requires a new frame every 17 milliseconds. Any network jitter of the same order results in additional delay or skipped frames, degrading the user experience. Additionally, these applications often require high data rates and continuously have data to send but can adapt their data rate based on congestion information from the network.
Strategies for integrating L4S with WMM
There are several approaches to integrating L4S with WMM. One strategy is to assign L4S traffic to a higher-priority WMM category. However, this must be done cautiously, as it can lead to significant asymmetries in data rates, potentially starving lower-priority traffic.
An alternative strategy is to use the same WMM category for all traffic, but one with optimized parameters. Using a single WMM category for all traffic—both L4S and non-L4S—can help balance channel access and reduce latency inflation due to contention. Hence, both L4S and non-L4S traffic would use the same channel access parameters (EDCA settings).
Figure 3 shows the measured performance when 1 (blue) and 2 stations (green) are connected to the same AP for different EDCA/WMM settings. All stations transmit L4S traffic and are assigned to the same WMM category.
Note that the peak latency (P99.9) is heavily inflated in the presence of multiple stations due to the contention for channel access. Unfortunately, using the video WMM category instead of the best effort doesn’t yield much improvement.
Figure 3: Wi-Fi latencies (round trip) for L4S traffic when 1 and 2 (blue and green) stations are connected to the same Wi-Fi AP and different WMM settings (best effort, video and a WMM category with L4S latency-optimized EDCA parameters).
Using L4S latency-optimized EDCA parameters, however, can significantly improve performance, as illustrated by the 3rd column in Figure 3. This approach reduces latency inflation due to contention five times (25 vs. 121 milliseconds) and even improves single-station latency by two times (14 vs. 27 milliseconds). While single-station scenarios penalized throughput slightly, multi-station setups either showed insignificant impact or even an increase in throughput with respect conventional approaches. These findings suggest that a single WMM category with optimized EDCA parameters could provide both low latency and high throughput for all applications.
The path forward for low-latency Wi-Fi
L4S has demonstrated its ability to virtually eliminate Wi-Fi queuing latency. However, to fully realize low-latency Wi-Fi’s promise, the MAC layer must be optimized. Combining L4S with WMM-based MAC optimizations can achieve substantial latency improvements in current Wi-Fi generations, such as Wi-Fi 6 and Wi-Fi 7.
Looking ahead, Wi-Fi 8, which is currently becoming standardized, presents an opportunity for even deeper optimizations in the MAC layer to deliver unmatched low-latency performance. These advancements are important as future applications demand higher data rates with minimal delay. To drive industry-wide engagement and innovation, Nokia Bell Labs presented these findings to the Wireless Broadband Alliance (WBA) in April 2024.
L4S technology is set to revolutionize Wi-Fi by delivering the low-latency performance required by modern applications. By addressing both queuing delay and the Wi-Fi MAC layer, we can unlock a future in which latency is no longer a bottleneck but a gateway to seamless, real-time digital experiences. As we continue to push the boundaries of what’s possible with Wi-Fi, L4S will play a crucial role in shaping the connected world of tomorrow.
For a more in-depth understanding of L4S, read our white paper.
Learn about our L4S testing with Excentis. Download the paper, Is L4S the real latency killer | Insights (excentis.com).