400G and the Economic Shannon Limit: Good news for network operators
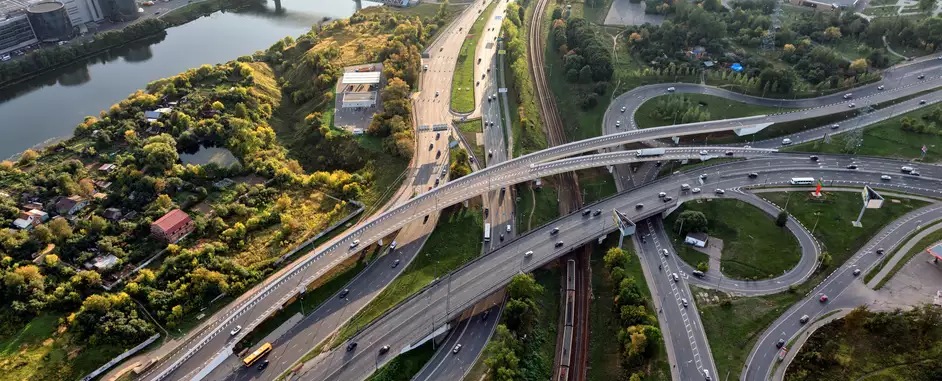
In a previous blog, I discussed how 400G is poised to become the universal network currency for years to come, but it's instructive to take a quick look back. A decade ago the iPhone was just taking off, and along with 4G mobile broadband it ushered in a new era of anytime, anywhere connectivity. 2010 also saw Netflix launch its streaming service internationally on its way to over 200 million subscribers. So it’s no surprise that bandwidth has continued to grow at roughly 30 percent per year, and with the permanent changes in remote work and video conferencing we're seeing as a result of COVID-19, this growth isn’t likely to slow down any time soon.
The year 2010 brought the introduction of 100 Gigabit Ethernet and coherent optical technology. Continuous innovations in coherent technology have steadily increased the capacity of optical systems, while pushing down the cost per bit transported. The result is that network operators have been able to accommodate the deluge of traffic while maintaining an effectively flat level of capital spend. This slow growth in CAPEX is critical to their ability to stay profitable in the face of stagnant subscriber revenue. As we enter the 400G era, the challenges for network operators are to continue to scale their networks, drive cost down, and optimize transport around 400 Gigabit Ethernet.
But there's a problem. The rapid (and dare I say easy) gains in capacity and cost reduction enabled by successive generations of coherent optics are largely behind us. Cost per bit reduction has been driven in large part by improvements in the spectral efficiency of coherent wavelengths. Shoving more bits into fewer interfaces and fewer fibers lowers costs. Yet spectral efficiency is famously bounded by the Shannon Limit. How close are we to this limit? Nobody knows for sure. There are undoubtedly still some gains to be had but perhaps that's not the question we should be asking. The more salient question might be this one: Does it make sense to continue to pursue gains in spectral efficiency, or would it be better to shift the focus of coherent research and development toward other methods of scale and cost containment? In other words, we may already have bumped up against what some call the Economic Shannon Limit.
The Economic Shannon Limit
Simply put, the Economic Shannon Limit means that further efforts to maximize spectral efficiency are unlikely to generate a better return on investment (lower costs for operators) than exploring alternative technological avenues. Does our reckoning with the Shannon Limit mean that coherent innovation will come to a stop? Certainly not. But it does mean that the focus of investment and innovation will shift from eking more spectral efficiency out of a fiber – or the related metric of achieving higher wavelength rates — to technologies that optimize power and space to better match coherent interfaces to specific applications, as well as maximizing interface reach for workhorse speeds like 400G. And this is exactly what's happening.
Each new generation of coherent technology has raised baud rates and lowered power per bit. Operation at higher baud rates increases the reach of a given wavelength rate. With spectral efficiency flatlining, this has become the primary benefit of new digital signal processor (DSP) generations. Operating at 90+ gigabaud, fifth-generation DSPs enable 400G rates over long-haul and subsea applications that span many thousands of kilometers, whereas two DSP generations ago, 400G wavelengths were limited to short metro spans of roughly 200 km or less. Nokia calls this 400G Anywhere, and our PSE-V Super Coherent (PSE-Vs) DSP is uniquely optimized around these demanding applications.
Investment in DCOs
Lower power per bit is enabled by new generations of silicon, which allows DSP engineers to cram more sophisticated signal processing and higher rates into the same power envelope. Alternatively, advances in silicon can be used to lower the absolute power of an interface from one coherent generation to the next. Both improvements reduce cost per bit, but the latter has given rise to smaller, more efficient application-optimized coherent interfaces, and network operators are already embracing them.
Nokia's third- and fourth-generation DSPs feature a compact variant to complement high-performance super-coherent DSPs. These variants offer a better fit for power- and cost-sensitive applications that do not demand the highest spectral efficiency. Despite their lower power, these compact optical interfaces have relied on board-mounted DSPs, most recently leveraging pluggable analog coherent optics, or ACOs, to house the optical front-end (OFE) components. With fifth-generation optics, this trend advances further to include the widespread adoption of digital coherent optics, or DCOs, where the DSP and optical front-end components are tightly integrated and packaged together into a discrete module.
DCOs can address two different applications. High-performance coherent optics – which traditionally require discrete board-mounted optics and DSPs – can be packaged into a DCO format. The tight integration of high-speed optics and electronics within a small form factor ensures optimal and consistent performance while facilitating integration into multiple platforms and line cards. Complementing these are pluggable DCOs packaged into standardized form factors such as QSFP-DD or CFP2. The big benefit of pluggable DCOs is their low power, small size and, most importantly, their portability (or pluggability) into a wide range of platforms that utilize standardized input/output (I/O) ports, including routers, switches and optical transport systems.
But DCOs demand significant expertise, investment and coordination across multiple disciplines to meet performance requirements within the strict power and space envelopes of standardized module form factors. Future investment will concentrate on this tight integration of electronics and optics – DSPs and silicon photonics. This is the focus of Nokia's WaveFabric Elements technology portfolio.
What DCOs mean to network operators
As fifth-generation optics become available, we’re seeing a multitude of interface types, with the majority packaged into a DCO. Initial steps toward application optimization in previous generations have now matured into a wide array of interfaces driven by performance on one end and by size and power on the other, plus options in between.
There is of course 400ZR, the standardized, pluggable, low-power interface that promises to dominate metro data center interconnect (DCI) and usher in a new era of router and switch-based pluggable optics. 400ZR+ is a marginally higher-performing version of ZR. It shares many of ZR’s characteristics, including pluggability, while adding features such as multi-rate capability.
400G Multihaul blends the best characteristics of high-performance and profile-optimized pluggable optics. A superset of 400ZR and ZR+ capabilities, it adds higher output power and service provider-oriented features such as OTN framing. A standardized pluggable form factor makes 400G Multihaul DCOs suitable for use in optical transport platforms as well as IP routers.
Pluggable DCOs offer numerous advantages to network operators, including:
- Pay-as-you-grow interfaces: Coherent optics represent a significant portion of network spend. The ability to defer expenditure until demand arises helps network operators, particularly when deploying dense, multi-port line cards.
- Optics upgradeability: Optical and IP platforms can take advantage of improved performance, new features and possibly higher rates as DCOs rapidly mature throughout the lifespan of the line card or platform that supports them.
- IP-optical coherent integration: As they are implemented into common router pluggable optics form factors, DCOs will usher in a new era of IP-optical integration by eliminating the density penalty previously associated with router-based optics.
- Multi-vendor optics: DCOs open up the possibility of procuring coherent optics from vendors other than the platform provider, although there are significant technological and operational hurdles that must be overcome before this becomes widely feasible.
These application-, power-, and density-optimized pluggable DCOs all have one thing in common – they are designed around 400G transport. As 400G will soon become the common currency of IP and optical networks, network operators will be able to choose among multiple interfaces to achieve the lowest cost per bit while simultaneously reaping the tremendous operational benefits that come with building an end-to-end 400G network across their IP and optical networks.
Opening up new possibilities
Pluggable DCOs aren't just about cost reduction. Their revolutionary power consumption, size and cost make possible applications that were previously ruled out as uneconomical or that were simply unfeasible because of power and space constraints. Sometimes reaching a limit opens up new possibilities. While we've yet to hit the physical Shannon Limit, diminishing technological returns have forced us to confront the Economic Shannon Limit and to steer precious R&D resources in a different direction. But the goals are still the same – to continually lower cost per bit, and to help network operators not just stay afloat but to innovate as they transform their networks for the 400G era.